Published in final edited form as:
Jumalyyev, Kerem, Maria Jahnert, & Anika Ayman. (2024).
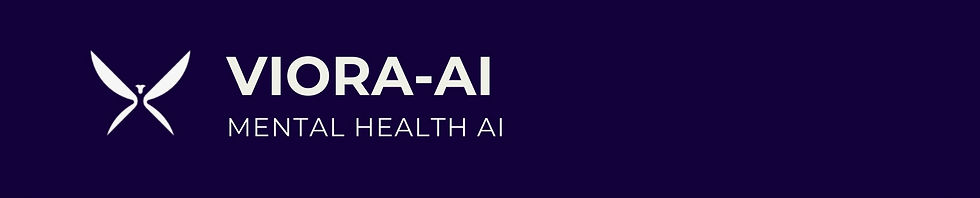
Maria Jahnert, MSc¹˒³; Ayman Anika²˒³; Kerem Jumalyyev³;
¹ The Rhenish Friedrich Wilhelm University of Bonn, Bonn, Germany
² Independent University, Bangladesh (IUB), Dhaka, Bangladesh
³ Viora-AI: Mental Health AI Co. , Berlin, Germany
Abstract
Brain organoids refer to miniature models of human brain tissues grown in vitro. These three-dimensional (3D) neural tissues are grown from stem cells and can be used to study the early stages of development of the human brain, test drugs – and by extension, learning capabilities – as organoids are essentially made from living cells. Developments in computational modelling have made it feasible to examine and stimulate brain organoid activity. Therefore, by utilising computational modelling, we can understand the learning capabilities of brain organoids. The article details the creation of computational models designed precisely for brain organoids. By analysing the challenges and future implications, the study discusses how computer models can bridge the gap between experimental biology and theoretical neuroscience.
Introduction
Often, many experts have described the human brain as the most complex of the universe. It is a labyrinth of neurons and made up of countless synaptic connections. Though researchers have been trying to understand its learning mechanism for years now, much remains to be understood and discovered. In this regard, brain organoids have displayed the potential to replicate the synaptic connections and neural activity of the human brain.
One research study, published in Nature Reviews Neurology, detailed that the three-dimensional human organoids offer a promising avenue to address the. limitations of traditional animal models by providing a closer approximation to human biology for disease modelling (Eichmüller & Knoblich, 2022).
Therefore, brain organoids can mimic key aspects of the brain. Now, scientists have started probing whether computational models can help us decode and in turn, improve the capabilities of organoids. A study has shown that computational models can indeed play a useful role by decoding the intricate dynamics of brain organoids – consequently, aiding researchers to simulate, predict, and interpret organoid behaviour (Pasca, 2019).
In this article, we explore two fundamental questions: What are brain organoids? Can
computational tools transform our understanding of brain organoids?
The Marvel of Brain Organoids: What Are They?
Generally, brain organoids are derived from stem cells, essentially from embryonic or induced pluripotent stem cells (iPSCs). Nevertheless, adult stem cells or fetus-derived cultures can be used in cases for some non-brain organoid modelling (Eichmüller & Knoblich, 2022). Over time, organoids are coaxed to differentiate into neural progenitor cells and these progenitors organise themselves into structures that resemble regions of the developing brain.
Unlike traditional cell cultures, functional neuronal networks along with spontaneous electrophysiological activity are exhibited by these organoids, and in some cases, responsiveness to external stimuli (Lancaster et al., 2013). All of these attributes of organoids suggest a semblance of network formation akin to a rudimentary brain.
The capacity of organoids for self-organisation raises important questions: How similar are organoids to human brains? Can they mimic learning processes through synaptic plasticity? Fundamentally, the process of learning in biological systems is based on the concepts of Hebbian plasticity, long-term potentiation (LTP), and long-term depression (LTD). By integrating data like multi-electrode array recordings from organoids' electrophysiological outputs, computational models can replicate these mechanisms.
The Role of Computational Models to Decode the Complexity
Decoding brain organoid activity is quite similar to navigating through a maze or mapping
uncharted territory. In this regard, computation models can offer a framework to translate
organoid behaviour, simulate learning processes, and make predictions that traditional
experimental setups alone cannot achieve.
That said, the computational models must regard the unique characteristics of organoids, such as their random growth patterns, partial differentiation, and variability in cellular composition. Recently, computational methodologies – for example, spiking neural networks (SNNs) and reservoir computing frameworks – have surfaced as effective approaches for simulating organoid learning. By replicating the plasticity-driven learning observed in experimental setups, these models can provide a virtual testbed for hypotheses (Trujillo et al., 2019).
Challenges in Computational Modelling
No two organoids are the same
Just like the human brain, organoids have distinct traits – they develop differently, leading to. variability in structure, size, and functionality. Hence, generating the ideal computational model that would be a “one-size-fits-all” is an immensely challenging task. As a solution, a research study suggests that computational models must incorporate probabilistic frameworks to address this heterogeneity (Qian et al., 2020).
Data scarcity
There are different technologies namely calcium imaging and multi-electrode arrays that
effectively capture organoid activity. However, the resolution and scale of these methods are limited so far. Often, computational models have to depend on data that is either incomplete or noisy, which requires the implementation of sophisticated pre-processing algorithms.
Are we creating consciousness?
The idea that brain organoids can display learning capabilities similar to human brains raises important ethical questions and philosophical debates about their potential rights. Can organoids inadvertently feign sentient experiences? As an apt answer to this question, a research study suggests ethical frameworks must evolve alongside technology to address these concerns (Farahany et al., 2021).
A New Frontier in Science and Ethics
Brain organoids are a marvel of science and developing computational models for the organoids showcases human ingenuity. The computational models can help us deepen our understanding of organoids – in turn human brain – and perhaps, can inspire noble innovations in AI and medicine.
However, the path ahead should be guided by both ethical and computational considerations. Interdisciplinary collaboration is needed, and only then, researchers can unveil the full potential of brain organoids to advance science and improve human well-being – while addressing the moral implications of their work equally.
References
Eichmüller, O. L., & Knoblich, J. A. (2022). Human cerebral organoids – a new tool for clinical neurology research. Nature Reviews Neurology, 18(11), 661–680.
Lancaster, M. A., et al. (2013). Cerebral organoids model human brain development. Nature, 501(7467), 373–379.
Pasca, S. P. (2019). The rise of three-dimensional human brain cultures. Nature Reviews Neuroscience, 20(10), 514–524.
Qian, X., et al. (2020). Brain-region-specific organoids using mini-bioreactors for modeling ZIKV exposure. Cell, 165(5), 1238–1254.
Trujillo, C. A., et al. (2019). Complex oscillatory waves emerging from cortical organoids model early human brain network development. Cell Stem Cell, 25(4), 558–569.
Farahany, N. A., et al. (2021). Ethical challenges in brain organoid research. Science, 373(6560), 10–15.
Comments